Comparative Analysis of Blood Flow within Regular and Stenosed Arteries: A CFD Approach
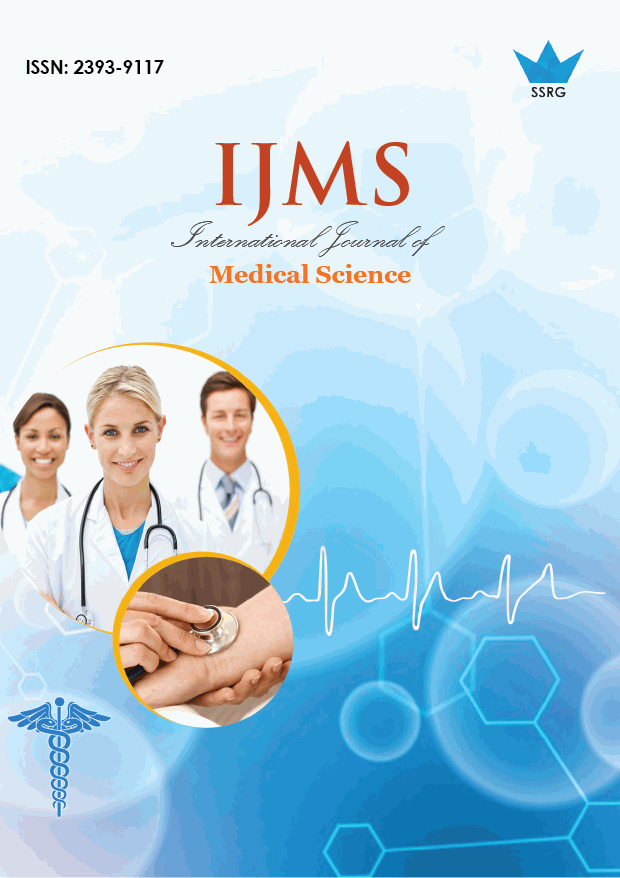
International Journal of Medical Science |
© 2019 by SSRG - IJMS Journal |
Volume 6 Issue 8 |
Year of Publication : 2019 |
Authors : Mohammed Nizam Uddin, Ahammad Hossain , Abdul Karim |
How to Cite?
Mohammed Nizam Uddin, Ahammad Hossain , Abdul Karim, "Comparative Analysis of Blood Flow within Regular and Stenosed Arteries: A CFD Approach," SSRG International Journal of Medical Science, vol. 6, no. 8, pp. 10-16, 2019. Crossref, https://doi.org/10.14445/23939117/IJMS-V6I8P102
Abstract:
It is of great physiological significance to simulate the behavior of diseased human arteries using computational methods, as it will help the clinicians in the early diagnoses of the disease. This paper reviews the comparison between the flow in a regular and a stenosed artery. It also reviews the application of Computational Fluid Dynamics (CFD), for simulating blood flow phenomenon in human arteries. The usage of the CFD in simulating and analyzing the anatomically realistic models are discussed. Various methodologies applied for assessing the effectiveness in predicting the behavior of blood flow in arteries is presented. The pressure gradient and flow velocities in the left coronary artery were measured and compared in the left coronary models with and without presence of plaques during cardiac cycle. Our results showed that the highest pressure gradient was observed in stenotic regions caused by the plaques. Low flow velocity areas were found at postplaque locations in the left circumflex andthe left anterior descending. There are direct correlations between coronary plaques and subsequent hemodynamic changes, based on the simulation of plaques in the realistic coronary models.
Keywords:
Blood, Coronary Artery, Stenosis, CFD, Hemodynamic, Wall Shear Stress.
References:
[1] Australian Institute of Health and Welfare, “The tenth biennial health report of the Australian Institute of Health and Welfare,” AIHW, Canberra, Australia, 2006.
[2] F. J. Rybicki, S. Melchionna, D. Mitsouras et al., “Prediction of coronary artery plaque progression and potential rupture from 320-detector row prospectively ECG-gated single heart beat CT angiography: lattice Boltzmann evaluation of endothelial shear stress,” International Journal of Cardiovascular Imaging, vol. 25, no. 2, pp. 289–299, 2009.
[3] S. K. Shanmugavelayudam, D. A. Rubenstein, and W. Yin, “Effect of geometrical assumptions on numerical modeling of coronary blood flow under normal and disease conditions,”Journal of Biomechanical Engineering, vol. 132, no. 6, article 061004, 2010.
[4] B. M. Johnston, P. R. Johnston, S. Corney, and D. Kilpatrick, “Non-Newtonian blood flow in human right coronary arteries: steady state simulations,” Journal of Biomechanics, vol. 37, no. 5, pp. 709–720, 2004.
[5] J. V. Soulis, T. M. Farmakis, G. D. Giannoglou, and G. E. Louridas, “Wall shear stress in normal left coronary artery tree,” Journal of Biomechanics, vol. 39, no. 4, pp. 742–749, 2006
[6] T. Chaichana, Z. Sun, and J. Jewkes, “Computation of hemodynamics in the left coronary artery with variable angulations,”Journal of Biomechanics, vol. 44, no. 10, pp. 1869–1878, 2011.
[7] Z. Sun, F. J. Dimpudus, J. Nugroho, and J. D. Adipranoto, “CT virtual intravascular endoscopy assessment of coronary artery plaques: a preliminary study,” European Journal of Radiology, vol. 75, no. 1, pp. e112–e119, 2010.
[8] Z. Sun, R. J. Winder, B. E. Kelly, P. K. Ellis, and D. G. Hirst, “CT virtual intravascular endoscopy of abdominal aortic aneurysms treated with suprarenal endovascular stent grafting,” Abdominal Imaging, vol. 28, no. 4, pp. 580–587, 2003.
[9] V. Fuster, “Lewis A. Conner memorial lecture: mechanisms leading to myocardial infarction: insights from studies of vascular biology,” Circulation, vol. 90, no. 4 I, pp. 2126–2146, 1994.
[10] X. He and D. N. Ku, “Flow in T-bifurcations: effect of the sharpness of the flow divider,” Biorheology, vol. 32, no. 4, pp. 447–458, 1995.
[11] W. Nichols and M. O’Rourke, McDonald’s Blood Flow in Arteries, Hodder Arnold, London, UK, 2005.
[12] S. Smith, The Scientist and Engineer’s Guide to Digital Signal Processing, Technical Publishing, Poway, Calif, USA, 1997.
[13] E.Wellnhofer, J. Osman, U. Kertzscher, K. Affeld, E. Fleck, and L. Goubergrits, “Flow simulation studies in coronary arteries- Impact of side-branches,” Atherosclerosis, vol. 213, no. 2, pp. 475–481, 2010.
[14] E. Boutsianis, H. Dave, T. Frauenfelder et al., “Computational simulation of intracoronary flow based on real coronary geometry,” European Journal of Cardio-thoracic Surgery, vol. 26, no. 2, pp. 248–256, 2004.
[15] Borghi, N. B.Wood, R. H.Mohiaddin, and X. Y. Xu, “Fluid-solid interaction simulation of flow and stress pattern in thoracoabdominalaneurysms: a patient-specific study,” Journal of Fluids and Structures, vol. 24, no. 2, pp. 270–280, 2008.
[16] W. W. Jeong and K. Rhee, “Effects of surface geometry and non-newtonian viscosity on the flow field in arterial stenoses,”Journal ofMechanical Science and Technology, vol. 23, no. 9, pp. 2424–2433, 2009.
[17] P. D. Ballyk, D. A. Steinman, and C. R. Ethier, “Simulation of non-newtonial blood flow in an end-to-side anastomosis,”Biorheology, vol. 31, no. 5, pp. 565–586, 1994.
[18] Zamir M, 2016. Hemo-Dynamics. Springer, New York.
[19] COMSOL Multiphysics Ltd. Version 4.3. Burlington. MA.