Closely Spaced Target Detection and Tracking under Spatial Ambiguity in Marine Surveillance Radar
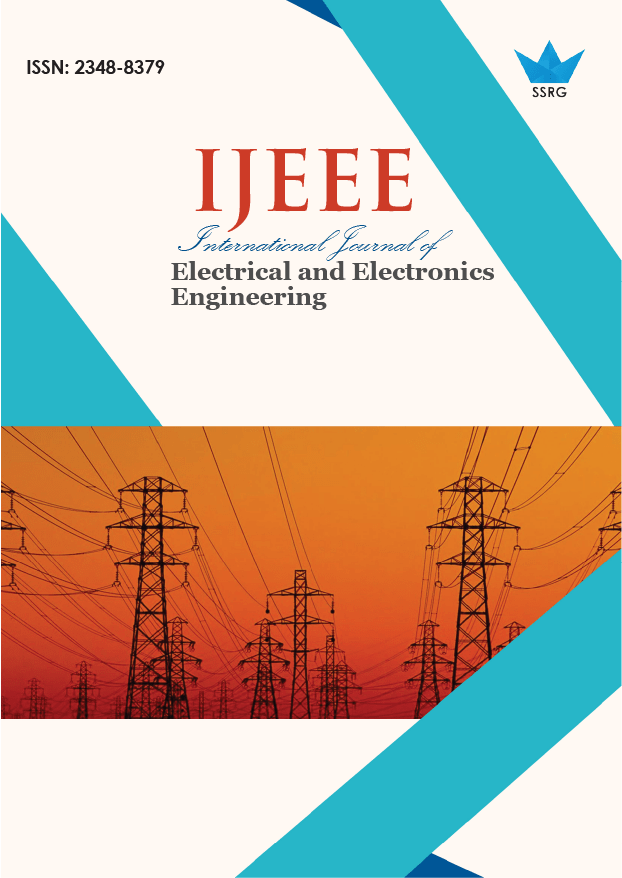
International Journal of Electrical and Electronics Engineering |
© 2023 by SSRG - IJEEE Journal |
Volume 10 Issue 9 |
Year of Publication : 2023 |
Authors : Navya R, Devaraju Ramakrishna, Sneha Sharma |
How to Cite?
Navya R, Devaraju Ramakrishna, Sneha Sharma, "Closely Spaced Target Detection and Tracking under Spatial Ambiguity in Marine Surveillance Radar," SSRG International Journal of Electrical and Electronics Engineering, vol. 10, no. 9, pp. 206-212, 2023. Crossref, https://doi.org/10.14445/23488379/IJEEE-V10I9P119
Abstract:
Target detection in radar is based on the strength of the received signal. Received echoes are processed to identify the target of interest by comparing the strength of the baseband signal to the surrounding noise. Radar processors will generate two plots for two targets if the radar can resolve both targets. Two closely spaced targets will fall within a beam resolution cell, which results in the generation of a single plot for both targets by radar. In this article, a new centroid algorithm based on the flattening and holding technique is proposed. The received sensor data is processed for each Azimuth Change Pulse (ACP) to identify all possible plots. At this point, the plotted intensity values for the detected targets are identified at individual azimuth angles. The proposed centroid algorithm consists of two stages. In the first phase, a unique averaging process is applied to smooth the intensity curve when two target azimuths have different intensity values. In the second stage, a dynamic threshold is computed and applied to the smoothed curve to identify and extract the original targets along the target spread in the ambiguity region.
Keywords:
Range and azimuth, Azimuth change pulse, Sensor resolution, Strength averaging, Dynamic threshold.
References:
[1] Yaolin Zhang et al., “Target Separation Detection and Motion Parameter Estimation Method Based on Time-Varying Autoregressive Model,” Journal of Engineering, vol. 2019, no. 21, pp. 8107-8110, 2019.
[CrossRef] [Google Scholar] [Publisher Link]
[2] Junhao Xie et al., “Tracking of Range and Azimuth for Continuous Imaging of Marine Target in Monopulse ISAR with Wideband Echoes,” International Journal of Antennas and Propagation, vol. 2016, pp. 1-17, 2016.
[CrossRef] [Google Scholar] [Publisher Link]
[3] HAN Xun, DU Lan, and LIU Hongwei, “Translation Compensation and Micro-Motion Feature Extraction of Space Cone-Shaped Target,” Chinese Journal of Radio Science, vol. 29, no. 5, pp. 815-820, 2014.
[CrossRef] [Google Scholar] [Publisher Link]
[4] Benjamin J. Slocumb, “Surveillance Radar Range-Bearing Centroid Processing,” Signal and Data Processing of Small Targets, vol. 4473, 2001.
[CrossRef] [Google Scholar] [Publisher Link]
[5] Muhamamd Ishfaq Hussain et al., “Multiple Objects Tracking Using Radar for Autonomous Driving,” 2020 IEEE International IOT, Electronics and Mechatronics Conference (IEMTRONICS), pp. 1-4, 2020.
[CrossRef] [Google Scholar] [Publisher Link]
[6] E.L. Cole et al., “Novel Accuracy and Resolution Algorithms for the Third Generation MTD,” National Radar Conference, pp. 41-47, 1986.
[Google Scholar]
[7] Ankith Manjunath et al., “Radar Based Object Detection and Tracking for Autonomous Driving,” 2018 IEEE MTT-S International Conference on Microwaves for Intelligent Mobility (ICMIM), pp. 1-4, 2018.
[CrossRef] [Google Scholar] [Publisher Link]
[8] Suresh Ponnan et al., “Extracting Micro-Doppler Radar Signatures from Rotating Targets Using Fourier-Bessel Transform and Time-Frequency Analysis,” IEEE Transactions on Geoscience and Remote Sensing, vol. 52, no. 6, pp. 3204-3210, 2014.
[CrossRef] [Google Scholar] [Publisher Link]
[9] Shengyuan Li et al., “Range-Angle Dependent Detection for FDA-MIMO Radar,” 2016 IEEE International Geoscience and Remote Sensing Symposium (IGARSS), pp. 6629-6632, 2016.
[CrossRef] [Google Scholar] [Publisher Link]
[10] Li Zhang et al., “Time-Frequency Imaging Algorithm for Highspeed Spinning Targets in Two Dimensions,” IET Radar, Sonar and Navigation, vol. 4, no. 6, pp. 806-817, 2010.
[CrossRef] [Google Scholar] [Publisher Link]
[11] Merrill I. Skolnik, Radar Handbook, 3rd ed., Mcgraw HILL, pp. 1-1352, 2008.
[Publisher Link]
[12] Mark A. Richards, Jim Scheer, and William A. Holm, Principles of Modern Radar, Volume I, Basic Principles, SciTech Publishing, pp. 782-786, 2010.
[Google Scholar] [Publisher Link]
[13] Philip Thompson, Matteo Nannini, and Rolf Scheiber, “Target Separation in SAR Image with the MUSIC Algorithm,” 2007 IEEE International Geoscience and Remote Sensing Symposium, pp. 468-471, 2007.
[CrossRef] [Google Scholar] [Publisher Link]
[14] A.H.A. Sharif, and I.G. Cumming, “Doppler Centroid Estimation for Azimuth-Offset SARS,” Proceedings of the IEEE 1995 National Aerospace and Electronics Conference, pp. 134-139, 1995.
[CrossRef] [Google Scholar] [Publisher Link]
[15] Peyton Z. Peebles, and R.S. Berkowitz, “Multiple-Target Monopulse Radar Processing Techniques,” IEEE Transactions on Aerospace and Electronic Systems, vol. 4, no. 6, pp. 845-854, 1968.
[CrossRef] [Google Scholar] [Publisher Link]
[16] Cai Chenxi et al., “Algorithm of Automatic Target-Position Acquisition for Long-Range Surveillance Radar,” Journal of Tsinghua University (Science and Technology), vol. 42, pp. 897- 900, 2002.
[Google Scholar] [Publisher Link]
[17] D. Curtis Schleher, Automatic Detection and Radar Data Processing, Dedham, The Artech Radar Library, pp. 1-660, 1980.
[Google Scholar] [Publisher Link]